Investigators' Blog

Feedback: Processes that change the thing that caused them
15 August 2024
This is the third of a series of posts on complexity. We’ll be exploring some of the ways that studying complex systems gives us a more nuanced way of understanding the world, how this is relevant to all our lives, and the unique contributions we can make to this new way of understanding the world from Aotearoa New Zealand.
We’ve all been there. Someone steps up to a microphone to speak, and there’s a little high-pitched ringing around the edges of their voice through the speakers. In a flash, this has become a loud squeal. Jolted in their seats, the audience clasp their hands to their ears until the person with the best reflexes manages to leap across to turn down the volume.
This is feedback. A microphone placed too close to a speaker picks up the sound from the speaker and amplifies it, then sends it back out of the same speaker. The microphone now picks up the louder sound, and amplifies it further. This reinforcing feedback happens rapidly, and before long we’re being assaulted by a high-pitched squeal.
We can also imagine a thermostat in a room. We set a preferred temperature, and allow the heating to run until this temperature is reached. At that point, the thermostat switches off the heating, and the room slowly cools. This is balancing feedback. Once the room has cooled below the set temperature, the heating is switched back on.
Feedback is all around us
Feedbacks are processes that change the thing that caused them.
Let’s say that we have a system with two parts: A and B. A is connected to B by one process, and B is connected to A by another. If the state of A changes, it triggers a corresponding change of some amount in B, because they are connected. But now, B has been modified, and because it is also connected to A by a second process, the change in B also results in further change to A.
The processes connecting A to B, and B to A, are both feedbacks, and those feedbacks can be reinforcing or balancing. Audio feedback and thermostats are classic examples of these two types of feedback. A reinforcing feedback drives further change in the direction a process was already going. It is a self-sustaining, or amplifying, feedback, which can lead to exponential growth or decline. A balancing feedback, on the other hand, leads to a reversal in the direction of change. So something that was increasing starts to decrease, and vice versa, which can lead to stability or equilibrium.
The concept of feedback is key to understanding complex systems in reality, reaching well beyond the scales of the examples of audio feedback or temperature control. Systems of feedback determine patterns we can see in the world around us. These patterns can be observed over time within any complex system, whether that be from the perspective of the earth sciences, biology, how cities work, or human health.
When we observe these dynamic patterns, whether it is exponential growth (like a virus spreading) or collapse (like the Wall Street crash of 1929) or even if a system is in a state of seeming stability, it is useful to explore the feedback processes behind these patterns. It is through understanding feedback that deep insight into fundamental causes and how to make change can be found.
Reinforcing and balancing feedback on a global scale
In studying the climate we can see reinforcing and balancing feedbacks playing out on a global scale. We know the release of carbon dioxide from burning fossil fuels results in more longwave radiation being trapped in our atmosphere, which leads to warming. This warming melts sea ice and thaws permafrost. Which in turn lead to further warming, which leads to more melting, and so on. This is reinforcing feedback, just like the squeal of a microphone.
Like the way a thermostat works, rainfall is an illustration of balancing feedback. Evaporating moisture from the surface of the Earth leads to increasing water vapour in the air. This increasing humidity then makes it harder for further water to evaporate from the land or ocean. Eventually, as the air becomes saturated, it rains, humidity is reduced, evaporation resumes, and the whole cycle starts again.
Feedbacks between people and environments
There are destructive inevitabilities, such as the increase in extreme weather events, of planetary system feedbacks like warming caused by too much carbon dioxide. But there are also constructive feedbacks where relationships between people, our practices and culture, and the built and natural environments are leading to new and innovative solutions.
One helpful reinforcing loop in the area of energy, where increasing demand for solar and wind power is driving down the cost of these clean energy sources so that they become more affordable for households.
In transport, if we can understand feedback processes underpinning car dependence, we can shift from reliance on cars to more people walking, wheeling and biking. For example, we know that fewer people ride bikes when they associate them with negative experiences of car danger and injury. If traffic on busy streets is slowed and road space is allocated to bike lanes, more and more people become visible, and you will see more people riding different kinds of bikes. When people see others like themselves on bikes having fun while getting around, this is a reinforcing feedback as they feel inspired and encouraged to then give it a go.
The ways we adapt to a changing climate bring new feedback processes into play. When people’s homes and land are threatened by severe weather, flooding, sea level rise and coastal erosion, balancing feedback responses – such as the construction of sea walls – might successfully buffer a worsening crisis in the short-term. But adaptations may not be sufficient in the long term and may in fact cause greater harm through attracting further housing development and therefore increasing the number of people exposed to climate dangers.
Understanding feedback is essential for finding solutions
Identifying and understanding processes of feedback is critical to understanding the complex causes behind the issues that we face. If we understand feedback, we can find more appropriate and sophisticated solutions, and become aware of dangers and unintended consequences. If researchers and policymakers incorporate feedback in their methods and practices, we can create more effective pathways of action that result in beneficial outcomes.
In the next post in this series on the foundations of complex systems, we’ll be taking a look at how we can understand phenomena across different scales in order to intervene more effectively.
A collaboration between Te Pūnaha Matatini Principal Investigators Alex Macmillan and Nick Golledge, and illustrator Hanna Breurkes. Edited by Jonathan Burgess.
Read more about the foundations of complex systems

Intervening in complex food systems to improve food security
29 July 2024
In certain areas of Australia, millions of sterile male fruit flies rain from the skies every two weeks. These Queensland fruit flies are reared to the peak of health in a special facility, then sterilised through irradiation, before being loaded into an aeroplane and dropped from the air. When the sterile males mate with local females, the females are unable to lay viable eggs.
This method effectively suppresses fruit fly populations, which cost Australian growers hundreds of millions of dollars a year in damaged fruit, pest control and lost market access opportunities. But is this too many sterile fruit flies to drop from the skies? TPM Whānau member Dr Tom Moore wants to know.
The Queensland fruit fly is an important pest of concern for Aotearoa New Zealand. Although there have been multiple detections in Aotearoa, the fly has not yet established a foothold. But this comes at a cost. In the most recent incursion, 11 male flies were caught on Auckland’s North Shore – at a cost of $18 million.
Tom is a quantitative ecologist who specialises in integrating scientific hypotheses into statistical models to address causal questions. He is particularly interested in understanding how invasive species establish themselves through data that spans across time and space. Aotearoa relies heavily on its biological resources, so this sort of agricultural research plays a pivotal role in our economic prosperity and shaping a sustainable future.
“Current methods of controlling fruit fly populations are effective,” says Tom, “but without a deeper understanding of the causal processes at work these could become less efficient due to future change, resulting in unnecessary costs.”
Tom has seed funding from Te Pūnaha Matatini to explore new methods of causal inference, which is the process of determining the independent effects of the individual parts that make up larger systems. Causal inference techniques drawing on interdisciplinary methods linking mathematics, computer science and statistics have become popular in other parts of the world, but are not yet widely used in Aotearoa.
Current statistical approaches can be limited in their ability to identify causal relationships. “It’s very common to collect a lot of data without a targeted question, chuck it in all in a model, and see what comes out the other side,” says Tom. “Richard McElreath calls this a ‘causal salad’. So while a certain model might make good predictions, it may be misleading in terms of causation, and unhelpful in planning interventions.”
Causal inference is a technique that considers how variables are related. This approach has been applied in other systems like economics, but is a new and developing technique in ecology. Developing causal models to support agricultural management decisions like the suppression of fruit flies has the potential to significantly improve our understanding of how to effectively intervene in these complex systems to improve food security in an uncertain future.
“By explicitly modelling cause and effect relationships in complex systems based on ecological theory,” Tom explains, “these techniques can evaluate if, and under what conditions, cause and effect relationships can be identified.” This project is an exciting opportunity to both develop complex systems theory, and to apply it to make a real difference to agriculture in Aotearoa.
Tom is working with a team including Te Pūnaha Matatini Principal Investigators Dr Will Godsoe and Dr Giulio Valentino Dalla Riva to develop a non-linear model of population dynamics using three years of weekly fruit fly trapping data.* They are also collaborating with entomologist Dr Lloyd Stringer from Plant and Food Research, and three colleagues from the from the University of Canterbury: statisticians Professor Elena Moltchanova and Dr Phillipp Wacker, and doctoral student in computational and applied mathematical sciences, Pooja Baburaj.
Tom and his team will then use this modelling approach to test out different biocontrol scenarios – exploring whether less frequent deployment of biocontrol measures can achieve the same population suppression. “If the model shows that you can reduce the frequency of biocontrol to monthly and the data looks the same, that’s a great outcome,” says Tom, “and would save a bit of money.”
The team is motivated to explore and showcase how causal inference can be used within the scientific process when defining hypotheses to generate more meaningful insights. Tom is especially excited about sharing this approach with his colleagues at Plant and Food Research and the broader research community, and hosting a workshop with Te Pūnaha Matatini colleagues to increase the capacity of the causal modelling of complex systems in Aotearoa. He hopes that this work will inspire interdisciplinary collaboration between agriculture, ecology and statistics.
Tom says that “we can ultimately use causal inference to predict where invasive species might spread, and how their population dynamics will manifest in a system, so that we can make evidence-based decisions on how to respond.”
“This is a way that we can solve real-world problems with innovative and evidence-based research.”
Illustrated by Chelsea Sullivan.
*This data was collected as part of the post factory pilot of SITplus fly production project (FF17001) that was funded by the Hort Frontiers Fruit Fly Fund, part of the Hort Frontiers strategic partnership initiative developed by Hort Innovation Australia, with co-investment from Macquarie University, New South Wales Department of Primary Industries, Plant and Food Research New Zealand, and contributions from the Australian government.

Emergence: How interactions create complexity from simplicity
26 July 2024
This is the second of a series of posts on complexity. We’ll be exploring some of the ways that studying complex systems gives us a more nuanced way of understanding the world, how this is relevant to all our lives, and the unique contributions we can make to this new way of understanding the world from Aotearoa New Zealand.
The Makarora River sparkles in a distinctive blue as it winds its way from the Southern Alps into Lake Wānaka. When it reaches Boiler Flat, it splits into shallow channels that flow around ever-shifting small islands in its gravel bed. This river is a braided river – an iconic feature of Te Waiponamu, the South Island of Aotearoa New Zealand.
It’s hard to imagine much life thriving among the floods, droughts, instability and tonnes of gravel that characterise braided rivers. But if we zoom out to consider their entire breadth, braided rivers support an immense diversity of life.
Braided rivers are complex systems made up of interweaving channels that change continuously as water flow shifts and deposits sediments. This creates a changing mosaic of habitats from pools, to fast-flowing channels, to islands, that are the result of interactions between flow, sediment, and organisms like plants.
The groups of species found in different parts of a braided river are constantly changing. Depending on environmental conditions and how recently it was ripped apart by a flood, a patch could be empty or comprise any combination of species. Within each of these patches, the species interact with each other in the form of competition for resources or predation.
A complex, tangled web of life holds things together across braided rivers.
What is emergence?
Surprisingly, when all these local patches of a braided river are considered together, the composition and diversity of species in the overall system tends to stabilise through time.
This stability is explained by ‘emergence’ – a key concept in complex systems. Emergence is a process we see everywhere, and occurs when small things interact to create larger things which also interact, behaving in new and unexpected ways.
The stability of whole braided rivers results from the complex interplay between physical and biological processes, feedbacks between different elements and systems, and their ability to self-organise. That is, the stability of the whole river system emerges from its unstable component parts.
Where else can we see emergence?
Complex systems cannot be described by simply adding together their parts. Instead, a higher property is made possible by the interactions or relationships operating within, and between, their parts.
Perhaps the poster child for all this is consciousness. This phenomenon has baffled scientists for centuries. Where does consciousness come from? How does it work? Although consciousness remains unexplained in many ways, scientists know that it emerges from complex interactions among a wide range of parts and processes in the brain.
We now know what the brain comprises, and how neurons – of which there are around 86 billion – work by sending electrical and chemical signals to each other. But consciousness itself remains unexplained, emerging from the interaction of neurons and the collective activity of this network in the brain. Like the whole braided river, consciousness cannot be reduced to any single neuron or neural circuit.
Emergence occurs in the social world as well. Every day, over eight billion people wake up, and go about the mundane tasks that take up most of our human lives. We get dressed, we eat, we imagine, we create, and we do what we can to survive. But our individual behaviour does not necessarily predict how societies function, because of the diverse connections and resulting complex feedbacks among humans.
We can see this in market economies where companies, civil society and governments interact. Prices and trends arise from the interaction of many buyers and sellers and government rules. These interactions can lead to outcomes and outputs not necessarily anticipated from a single exchange.
Although these interactions can have positive outcomes, such as efficient resource allocation, innovation, and overall economic welfare, they can also be undesirable. The 2007-08 Global Financial Crisis provides an example of this. In this case, emergent behaviours within financial markets, driven by complex interactions and systemic risks, led to substantial global economic and financial disruption that was largely unanticipated.
Emergence is one of the key processes that explains how our world works
Emergence is about how complex patterns, structures, and behaviours arise from interactions – giving rise to new system properties not found in the individual components alone.
In the next post in this series on the foundations of complex systems, we’ll be taking a look at how systems self-organise, adapt and evolve over time through a process known as feedback.
A collaboration between Te Pūnaha Matatini Principal Investigators Jonathan Tonkin and Julia Talbot-Jones, and illustrator Hanna Breurkes. Edited by Jonathan Burgess.
Read more about the foundations of complex systems

Caring for our earthly kin
11 July 2024
A collaboration between environmental geographer Emma Sharp and illustrator Jean Donaldson. Edited by Anna Brown and Jonathan Burgess.
Soil is complex. Beautiful. Wondrous. It gives us food, foundations and filters the air we breathe and water we drink.
In a very literal way, soil is also a part of us. Our bodies are not discrete entities. Our bodies are networks; we are but an assemblage of a host organism with microbial communities living in and around us. Our interdependencies with the soil we eat make for healthy microbial communities of our gut, of ourselves. Our insides are the outsides — the world.
Understanding soil, or land, as ‘kin’ is not a new concept in Indigenous knowing of the world, providing a perspective on the responsibility of treating, nurturing and caring for it as one would a relation. Kaupapa Māori researcher, activist and grower Jessica Hutchings has proposed that in our relationships with land, or soil, reciprocity is vital. This is understood and practised in some communities. It might seem a stretch to others.
Is ‘legal personhood’ a tool or a distraction for Māori relationships with nature? – Mongabay
If we accept that soil is part of us, then we must admit that we are at war with ourselves. We see this in political decisions to develop prime agricultural soils through ‘financialising’ land. We see it in national investment into intensive agrichemical use, and its endorsement through lax regulations. And we find it in individual behaviours of everyday (over)consumption of plastics, of wasted food, of the marketing of unnecessary convenience-chemistry that means that our soils bear the legacy of contamination.
The soil in our landfills, and what leaches out of them, tells us a lot about what our society really cares (or doesn’t care) about.
A drive for profit-focussed empire-building and an obsession with technological futurism has led us from sustainable ways of living with our environment to modern-day purposeful destruction of nature. This shows up in soil as erosion, depletion, dust bowls, contamination, or suffocation.
Aotearoa New Zealand, while branding ourselves as natural, has constructed an identity around producing off land that is dependent on pesticides. New Zealand does not keep track of chemical use and release. We don’t have decent monitoring in place to understand the extent of the problem.
Humanity has exceeded planetary boundaries, threatening the safe operating space for humans, and for non-human others. We have entered a period of triple planetary crisis: of biodiversity loss, pollution and climate change, all of which have a bearing on the state of our soils.
Outside the safe operating space of the planetary boundary for novel entities – Environmental Science & Technology
Humans have caused this, so humans can change this.
It requires bold, brave leadership to do what is ethical, care-full and in the interests of our common planetary future: to call for environmental disarmament, to dismantle infrastructures of violence against nature.
We might (re)value soil in ways that mobilise what we think about as our ethical responsibilities to and in the world. These ethics recognise the complexities of the communities in and of soil: human and microbe, food-waste collector and kaimahi māra kai, invertebrate and farmer. These complexities can never be reduced down to a calculus of yield and profit.
So, let’s notice our soils. This might be in exploring the beauty of fungi, in growing food, or on a walk in the ngāhere to breathe the sweetened air and mood boosting, ‘friendly’ bacteria.
Identification of an immune-responsive mesolimbocortical serotonergic system: Potential role in regulation of emotional behavior – Neuroscience
Slowing down to attune ourselves to the natural rhythms and temporalities of soil is effective, and it is affective: it changes us, and it changes our capacity to be in the world. It changes our imaginations of what a cared-for soil world might look like. Let’s then live with a soil ethic that asks not what if we nurtured our soils, but rather as if we did, by performing a politics of soil care, and enacting – making – a different world.
Emma Sharp is a principal investigator with Te Pūnaha Matatini who works on soil and food politics, care and community economies at Waipapa Taumata Rau the University of Auckland.
Jean Donaldson is a designer and native bird fanatic based in Te Whanganui-a-Tara. You can see more of her work at https://jeanmanudesign.com/.

Yes, you should mention the myth when you correct it
14 June 2024
A collaboration between doctoral researcher Laura Kranz and Illustrator Jean Donaldson. Edited by Jonathan Burgess.
You’ve probably had to study for a test or exam at some point in your life. When faced with a lot of material, how do you go about learning it? Perhaps you listen to it through your headphones while commuting because you’re an auditory learner.
But are you?
The idea that everybody has a specific learning style (visual, auditory, or kinaesthetic) is remarkably persistent. It seems to make sense that people learn best when they study information in their given learning style. However, there’s no evidence to support learning styles. Hundreds of studies in cognitive science show that people learn best when we receive new information in multiple ways, but that people don’t learn any better in their preferred style.
If you believe that learning styles are a thing, you’re not alone. Learning styles is a pervasive myth – in my research, more than 95% of people believe it to be true. We are taught it in schools, and unless you’ve specifically researched it yourself, or have been told it’s a myth, you have no reason not to believe it to be true.
People believe many false ideas. Most are pretty benign – it makes minimal difference to society if I believe that we swallow eight spiders a year in our sleep or that chewing gum stays in your stomach for seven years if you swallow it. But some false beliefs can have harmful impacts, like reduced vaccination rates or increased greenhouse gases. We all benefit from correcting myths that cause harm.
Attempting to replace belief in myths with correct information can be frustrating, but there are strategies that can help. One recommendation from research – including my own – is to mention a myth when debunking it. By mentioning a myth you are encouraging people to mentally link the pre-existing false belief with the correct information.
When people believe a false idea, they have a representation of this in their brain. When they are told new information that counters a pre-existing belief, the new information doesn’t simply erase and replace the old belief. Instead the new information forms its own, independent, representation in the brain.
Even if people believe the new information, the old false information is still around and can continue to influence thinking and behaviour. If somebody has a mental link between a false belief and a correction then when they bring to mind the false belief they are more likely to bring to mind the correction too. This reduces the likelihood that they use the false information to guide thinking and behaviour.
In my research I look at how to effectively correct scientific misinformation – both controversial myths (such as myths about vaccines) and non-controversial myths (such as myths about learning styles). We used to think that mentioning a myth when debunking it could be harmful. We know that people use familiarity as a proxy for truth – that is, the more familiar an idea feels to a person, the more likely they are to believe it to be true.
By mentioning a myth when debunking it we thought we might inadvertently strengthen belief in the myth by boosting its familiarity. However, it turns out that the relative benefit of mentally linking the myth and correction overrides any potential familiarity effect. This looks to be the case for both controversial and non-controversial myths.
Even when people don’t shift their beliefs to be in line with new information, people don’t tend to show increased belief in a myth when it is mentioned in debunking efforts. This is great news for science communicators: you don’t need to avoid mentioning a myth when debunking it.
Laura Kranz is a Te Tira Maurikura member who has been exploring the role of cognition and emotion in science communication for her doctoral research.
Jean Donaldson is a designer and native bird fanatic based in Te Whanganui-a-Tara. You can see more of her work at https://jeanmanudesign.com/.

Complex is different from complicated, and why that matters
5 June 2024
This is the first of a series of posts on complexity. We’ll be exploring some of the ways that studying complex systems gives us a more nuanced way of understanding the world, how this is relevant to all our lives, and the unique contributions we can make to this new way of understanding the world from Aotearoa New Zealand.
An aeroplane is pretty complicated. The large commercial planes that criss-cross our skies are made up of millions of parts, each one carefully designed and engineered. There are hundreds of years of scientific enquiry behind these machines that lift us off the ground and transport us at tremendous speeds around the world.
Each part of an aeroplane has a particular purpose, and contributes to achieving flight. You can reduce an aeroplane to the parts that make it up, and you can reduce flight to four basic principles: lift, weight, drag and thrust.
Aeroplanes are a great example of understanding the world by breaking it down into parts, and then understanding the whole system as the sum of these parts. Over the last few centuries, scientists have gotten pretty good at this approach.
But if you took an aeroplane apart, it would just be a big pile of parts again. Without a team of humans who understand how to rebuild it, this pile of parts would continue being a big pile of parts, rather than an aeroplane.
An aeroplane is very complicated, but it’s not complex. In the 21st century, as human societies are growing, becoming more interconnected, and having an increasing influence on planetary systems, it’s complexity that we need to understand.
On planet Earth, we’re surrounded by big piles of water. These big piles of water are made up of water molecules. As these molecules are heated, they rise up into the sky, and form clouds as they cool. They travel around the world, and become mist, fog, rain, snow and hail. They coat mountaintops, and they feed plants. They carve out landscapes, and they flow down rivers and back into the sea.
Where aeroplane parts create aeroplanes, water molecules are one of the components that create weather, and our climate. Weather is different from an aeroplane because it doesn’t need anything external – like a group of aeroplane engineers – to create it. This is why weather is a complex system, and an aeroplane isn’t. And where aeroplanes are reasonably consistent, the weather is never the same. Those piles of water molecules interact with each other and other elements of the weather system to produce infinitely different weather patterns.
Complex systems are all around you. And you as a human have arisen through the behaviour of complex systems. Biological cells organise themselves into animals and plants, and can create wondrous structures like the human brain.
When biological cells have self-organised into humans, those humans create societies, and those societies interact with the systems around them to influence things like the climate system, which in turn influences those societies. Everyone and everything exists within this interwoven system of systems with complex relationships that feed back and influence each other. These interwoven systems challenge our common understanding of cause and effect.
When Cyclone Gabrielle ravaged the local environment in Aotearoa New Zealand in 2023, the complexity of the situation became strikingly evident. Many factors determined the extent of the damage and the effectiveness of recovery efforts, from the interactions of atmospheric conditions to the vulnerabilities of built environments and the different ways that communities reacted.
Where wind patterns, topography, infrastructure resilience, and human actions and decision-making interact, they give rise to outcomes that cannot be predicted by analysing each component in isolation. We call this emergence, an important process resulting from the relationships within complex systems.
A better understanding of complex systems can help us to see the whole context of situations like extreme weather events, including their emergent properties. This will help us to better predict, understand and act when disasters strike.
To understand complex systems, breaking them down into their individual bits doesn’t cut it, as it is the relationships between the parts that have the most influence.
The challenges that we face in the twenty-first century are complex. The interrelated problems of economic crises, social inequality, food insecurity, migration, urban and rural living conditions, and globalisation have shown us the limitations of understanding the world as only complicated.
Although we have become very good at understanding and building the complicated, we have more work to do on understanding and acting on the complex. How does anything, like disease, information, and cultural practices, spread through systems?
Over the last century or so, the study of complex systems has given us a detailed way of understanding our world. We have learned a lot about how complex systems behave which can assist prediction and change. We have learned that how they evolve is quite similar across many different areas, and that understanding one system can give surprising insights into another. As we have learned more about complex systems behaviour, we have also learned more about how this behaviour is tied together through theories of complexity that enable structures and processes to be defined, detected and studied.
As humans grow as an influential force on Earth and beyond, we need to better understand our relationships with each other and with the systems that we encounter. The developing field of complex systems is giving us the concepts, language, and tools to do this. In this blog series, we will explore some of the foundations of complex systems and how they can help us to understand our world.
In the next post in this series on the foundations of complex systems, we’ll be taking a look at emergence – when small things interact to create larger things which also interact, behaving in new and unexpected ways.
A collaboration between Te Pūnaha Matatini Principal Investigators Anna Matheson and Markus Luczak-Roesch, and illustrator Hanna Breurkes. Edited by Jonathan Burgess.
Read more about the foundations of complex systems
Systems and people: Te Pūnaha Matatini submission to the Science System Advisory Group
29 May 2024
Associate Professor Priscilla Wehi and Professor Markus Luczak-Roesch
Co-Directors, Te Pūnaha Matatini
We appreciate the opportunity to provide input into the work of the Science System Advisory Group (SSAG) on the review of the research, science and innovation system (RSIS) in Aotearoa New Zealand. We provide this input in our capacity as co-directors of Te Pūnaha Matatini – Aotearoa New Zealand’s Centre of Research Excellence for Complex Systems.
Because there has already been significant consultation in recent years about the RSIS (especially in the context of Te Ara Paerangi, to which we provided a comprehensive and evidence-backed submission), we want to keep this submission to the SSAG concise and focused on two recommendations:
- Take a systems approach to the evaluation and re-design of the RSIS.
- Put the people within the RSIS at the centre of attention.
Take a systems approach to the evaluation and re-design of the RSIS
Adopting a systems approach to reforming the RSIS should involve a comprehensive and integrated method that considers all components and their interactions within the research ecosystem and of the research ecosystem with the wider society. This approach should ensure that the reform is holistically forward-thinking and give consideration to the need to restore the credibility of research and science in an increasingly polarised society.
A critical step in a systems approach should be the identification of levers of change within the current RSIS that are expected to be effective for a transition into a desired future state. However, our appeal is for any transition to happen with great care by first evaluating implications and impact of change to avoid creating, perpetuating or amplifying inequities in the RSIS. This should be backed by robust, high-quality evidence.
Put the people within the RSIS at the centre of attention
As a Centre of Research Excellence (CoRE) we have a particular perspective on the lived reality of people within the RSIS that other organisations may not experience as directly as we do. We are attached to a host institution (the University of Auckland), but we are not a legal entity. We don’t employ researchers, but we build researcher networks and support some of the research activities of the people within our networks through research funding.
We have experienced – specifically over the last five years – a diversification of our principal investigators’ professional circumstances. It is much more common now that some of Aotearoa New Zealand’s best researchers are not permanently employed by ‘traditional’ organisations with a mandate to undertake research (e.g., universities or Crown Research Institutes (CRIs)). Instead, we much more frequently see mixed employment (part-time with a traditional organisation and part-time with a private organisation) as well as scholars who have no link to traditional research organisations (i.e., they work as independent scholars or are employed by a private research organisation). However, involving people from outside of traditional organisations in CoRE-funded research is not straightforward due to structural, procedural and financial misalignments (e.g., independent scholars and scholars in private organisations aren’t usually covered by a base salary to attend CoRE events and, therefore, need to be compensated on a per day basis or invest their time in events for free).
Furthermore, because these scholars have (partially) left the traditional academic research system, they are no longer incentivised to publish their research in academic outlets. This is in direct conflict with the expectations from many research funders (including the Tertiary Education Commission (TEC) that funds CoREs) that evaluate research success and impact (proposed and achieved) through the proxy of publications in peer-reviewed academic outlets.
We want to emphasise that we think the diversity of researchers’ employment circumstances should be considered a feature (and not a flaw) of a modern RSIS that is committed to what is often referred to as the “third mission” (specifically in central Europe). The “third mission” refers to the interweaving of universities and research organisations with society, municipalities, and the economy. We hope the SSAG will make recommendations that allow for larger flexibility in how research funding can be used to foster this mission and enable wider participation by people in research activities independent from their individual organisational affiliation. Associated with this, we would also like to see effort put into communicating the value of research to societal groups and organisations.
We therefore reiterate that we would like to see careful assessment of the current research system based on robust work from a structural perspective, including current researcher ‘homes’ and employment destinations for both domestic and international students, and an assessment of the necessary requirements to create and maintain a stable, flourishing workforce.
Systems and people: Systems and people: Te Pūnaha Matatini submission to the Science System Advisory Group [PDF 658KB]

The surprising benefits of planting trees on farms
24 May 2024
A collaboration between bioeconomy expert Sandra Velarde and illustrator Jean Donaldson. Edited by Jonathan Burgess.
Kyle Wills is not afraid of planting trees on his beef and deer farm.
Kyle is a farm advisor from Central Otago. In the winter of 2022 and 2023 he planted over 7,000 trees in two different areas of his own farm. One area was unimproved native pasture and the other was a very exposed developed paddock that was not performing.
He has planted a variety of trees such as oaks, eucalyptus, alders, poplar, tagasaste, mulberry, pears, apples, apricots and plum trees.
These trees will be increasingly important on Kyle’s farm. The number of hotter days in summer in the next 20-70 years is expected to double in Te Wai Pounamu, the South Island of Aotearoa New Zealand. Cows without shade or shelter on site may suffer heat stress, and this affects the amount of milk that they produce. Winter days may become wetter as well, and it can get pretty windy out there in places like the Canterbury Plains.
Farmers care for their animals, and as the weather becomes more extreme, they will need more protection. Trees give them this protection. This is just one of the numerous benefits of planting trees on farmland, which is called agroforestry.
An inspiration for other farmers, and an easy way to get started
One day, a nearby farmer saw what Kyle was doing with the trees on his farm. She asked Kyle to design a similar system for her. She was concerned with future potential reductions of water to irrigate her sheep and beef farm and a lack of shelter for her livestock. Combined with low rainfall, poor soil water holding capacity, and exposure to winds and hot summers, this farmer liked the idea of planting some trees to improve the farm microclimate, and add other benefits such as creating feed for grazing animals and income from carbon sequestration.
When temperatures rise, summers become hotter, winters wetter, and water permits may become uncertain, trees may be a way to build resilience on farms.
If you’ve ever driven through or flown over the South Island, you might have noticed vivid green circles amongst the fields. These are irrigation circles, created by centre pivot irrigators – hulking metal spans that spray water across the fields as they trundle around in a circle.
The dry corners created by this approach to farming are an easy place to start with planting trees. If you put them all together, there are about 35,000 hectares of these corners in Canterbury, ripe for tree planting.
Word gets around
In his work as a farm advisor, Kyle spent a lot of time talking to farmers from Claxby Farms and Ngāi Tahu in Canterbury about planting trees on their farms. He has spent hours visiting and listening to farmers’ key concerns, values and farming objectives. Then he started looking for the trees that could grow on each farm to fulfil their different objectives.
Both farms cared about short term profitability but their long-term biodiversity goals are different. On their Hamua farm, Ngāi Tahu were willing to accept higher costs for establishment, utilising more fencing to create a space where native species could germinate if conditions allowed. Additionally, there were native species in the planting mix and the plan is to replace exotic trees with natives as they mature over time. This way, they would achieve their long term biodiversity aspiration, increasing the mana of te taiao.
Indigenous trees are natural magnets for native biodiversity. They behave as islands for indigenous flora and fauna. As pollinators and seed eating birds visit the trees for food, shelter or nesting places, indigenous trees encourage native afforestation. Two native species were selected for the agroforestry system on this farm: kōwhai and ribbonwood.
Kyle and the farmers tested some ideas face to face and on screen maps, with different layouts, tree species and shading rates. The farmers were full of questions:
- How will pasture production be affected if I put in trees in dryland corners?
- Which trees will create food for the animals or not cause any problems?
- Will I be able to get income from the trees without harvesting them?
- How much carbon will the trees sequester?
Together, they designed an agroforestry system that is both good for the environment and potentially profitable. Trees on the dry corners of irrigated dairy farms can reduce nutrient leaching, sequester carbon and keep the cows happy by providing food, shade and shelter.
A new era of agroforestry
In Aotearoa, past agroforestry experiences have not been positive. Previous approaches to planting trees on farms have been mainly based around pine trees for timber. This system was not designed with the farmer in mind, and was a little bit unimaginative.
These days we focus on farmers, and respond to their values and needs. We get out on to farms, talk to people, and listen intentionally to their needs. For someone like me, who has worked in agroforestry for decades, this is how the magic happens.
Putting trees back on farms is an exciting opportunity to create a resilient rural landscape for the future.
- Check out the research behind this story
- Take a tour of agroforestry systems within irrigated dairy farms in Waimakariri
Thank you to my colleague and farm advisor Kyle Wills for showing me how to get out of the books and make the magic happen, and to Cheryl Palm, one of my early agroforestry mentors whose legacy will live on with all the young scientists she trained. – Sandra
Sandra Velarde works on bioeconomy innovation and alternative forestry at WSP and is a Principal Investigator with Te Pūnaha Matatini.
Jean Donaldson is a designer and native bird fanatic based in Te Whanganui-a-Tara. You can see more of her work at https://jeanmanudesign.com/.
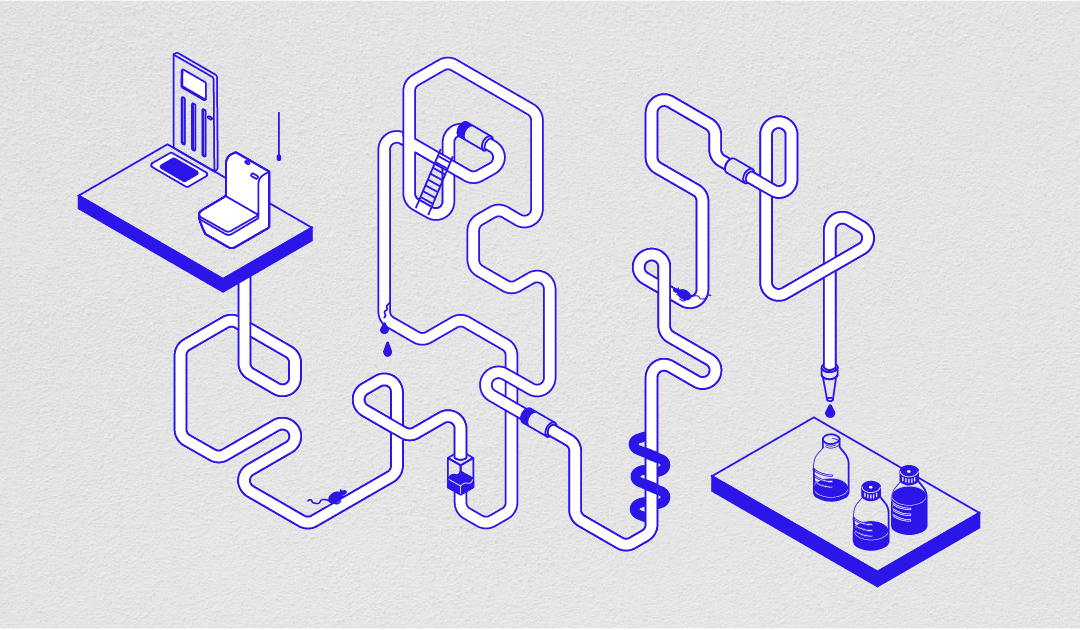
Every flush a donation to science
8 April 2024
A collaboration between analytical chemist Lisa Pilkington and illustrator Jean Donaldson. Edited by Anna Brown and Jonathan Burgess.
You probably don’t give a second thought to what you flush down the toilet. But wastewater and all that it contains provides valuable knowledge to researchers working in wastewater-based epidemiology.
Wastewater is everything you put down the drain – including number ones and number twos. Every time you rinse something down the sink, wash your clothes, have a shower or go to the bathroom, the wastewater flows into sewage pipes and travels to a wastewater treatment plant to be cleaned up before it’s allowed to enter waterways.
Researchers working in wastewater-based epidemiology analyse wastewater to find particular chemical compounds or ‘biomarkers’ that can give them information about a population’s exposure to or consumption of certain substances or microorganisms.
During the height of the Covid-19 pandemic, you might have heard about the analysis of wastewater to identify infections in individual communities. Wastewater-based epidemiology became an essential tool that helped scientists and the people making decisions about our health understand and monitor the progression of Covid-19 in the community. This type of investigation has most commonly been used to understand consumption of illicit drugs in populations – something that it is difficult to obtain sales data on!
We have recently applied this method to understanding alcohol consumption in our communities. In 2021 my team led one of the most comprehensive studies on alcohol usage ever conducted globally. Our six-month long study looked at alcohol usage in approximately 40% of Aotearoa’s population. It certainly gave some fascinating insights into our drinking habits.
But before we get to those – how do we analyse alcohol consumption using wastewater-based epidemiology? Let’s go through the journey of a biomarker. When you have that pre-dinner gin and tonic, frosty pint at the neighbourhood brewery or pinot noir on the couch after work, ethanol is the key ingredient. Our liver changes ethanol into a range of other compounds, including one called ethyl sulphate – the biomarker that we looked for in our work.
These compounds are then excreted in urine and flushed into the wastewater system, ending up at your local wastewater treatment plant. It is at the inlet of this treatment plant that we collect wastewater samples and take them to the lab for analysis. We measure the amount of ethyl sulphate in the samples and then use this value in calculations to work out the amount of alcohol consumed per person in the wastewater treatment plant catchment area.
Wastewater-based epidemiology has a lot of advantages over existing methods such as surveys and sales data in understanding a population’s consumption habits. It is unbiased, non-invasive, informative, low cost and near real time. Even when survey and sales data is available, wastewater-based epidemiology can fill gaps and provide depth and nuance to our understanding of consumption habits. This was certainly true for our study of alcohol in Aotearoa.
Some of the results of our study were fairly unsurprising. They showed that in Aotearoa, we consume more alcohol on the weekends compared to weekdays, more on long weekends compared to normal weekends, and during lockdowns all of these trends went out the window!
However, this study also unveiled some surprising trends that we didn’t anticipate:
- Larger cities have lower levels of alcohol consumption.
- On average, New Zealanders consume less alcohol than many other countries, including Australia, the United Kingdom, Canada and the United States of America.
- Drinking levels are higher in the South Island compared to the North Island.
- Spikes in consumption could be mapped back to particular events happening on a given day, including a one-night-only male revue show, ‘The Stallions’, performed in Queenstown.
The results of this investigation not only highlight the detailed level of information that can be obtained using wastewater-based epidemiology, but also show how useful this approach can be to inform organisations and help direct resources where they’re needed the most. Looking to the future, I have two different, but equally as important studies in the pipeline. The first is understanding vaping habits in Aotearoa. The second is investigating whether we can extend the techniques we have developed to monitor cancer prevalence in our communities.
So next time you flush, take a moment to congratulate yourself on contributing to research that can make a positive change to our communities.
Lisa Pilkington is an analytical chemist at Waipapa Taumata Rau, the University of Auckland, and a principal investigator with Te Pūnaha Matatini. You can read more about the results of the alcohol consumption in Aotearoa study here: https://doi.org/10.1002/asia.202301120.
Jean Donaldson is a designer and native bird fanatic based in Te Whanganui-a-Tara. You can see more of her work at https://jeanmanudesign.com/.
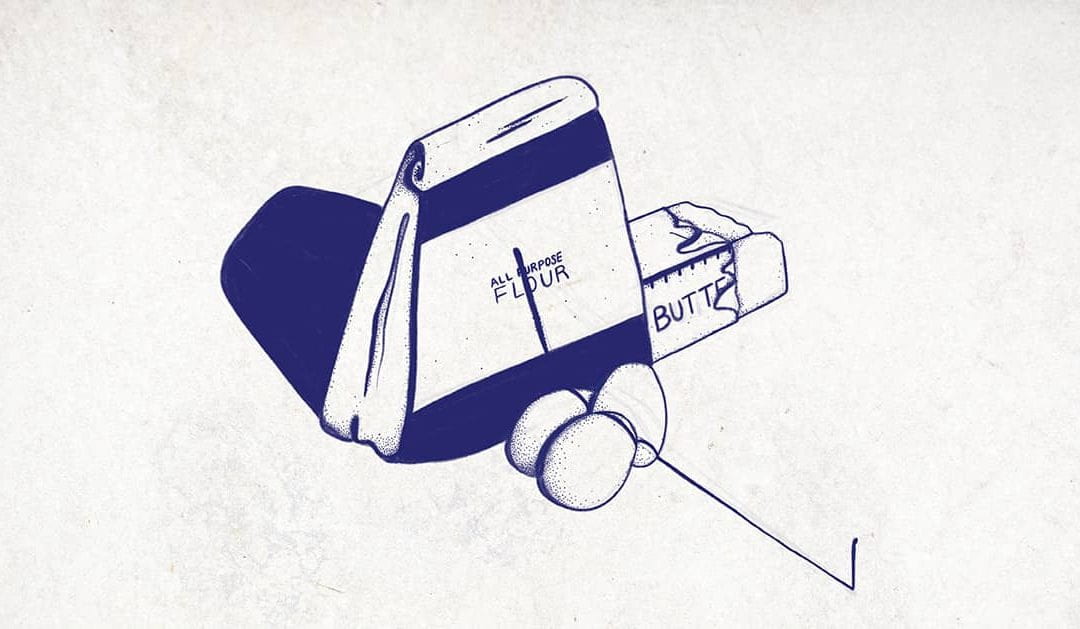
Sifting through the unknown with recipe-free research
I’m a baker, so I like having a recipe to follow. It’s comforting to have a set of ingredients and a list of instructions to start with — even if I treat the recipe as more of a guide than directions for a science experiment. Knowing where to start, and what I will most likely end up with at the finish helps me feel like I know what I’m doing, and means new recipes still have a sense of familiarity to them.
Research, or other projects, are similar. We generally have an idea of what ingredients and equipment we need, along with a recipe — or plan — of what we need to do to reach the outcome at the end. These plans are comfortable and familiar. They help us feel like we’re on the right track, and even when things don’t go as expected, we can figure out where things are different from the original plan, and either solve them or make a new plan from there.
Co-production is not like that.
In my experience so far, co-production is more like one of those team challenges in a MasterChef bake off — they’ve asked you to make a selection of items for afternoon tea and left it at that. Except you also don’t know who’s in your team, and your test kitchen hasn’t been kitted out. So before you can start, you need to figure out who’s in the team and what equipment you might need or have available — partway through you might also discover you’re actually in a field kitchen rather than the test kitchen!
Making a selection of items for afternoon tea sounds clear enough, but when you start thinking about it, you realise how many possibilities there are. Using co-production to create a selection of research questions about women’s health that are a priority to women is similarly broad. We’ve had to grapple with questions such as “Is women’s health all health issues that impact women?” or “Is it only those health issues that just women experience?”
When you don’t have a clear recipe to follow, it can be pretty hard working out where to start — the ingredients you use are influenced by what equipment you have available, and vice versa.
Thankfully, unlike a MasterChef challenge, we don’t have a ridiculously tight time limit. We’ve been able to take our time getting to know each other in the core research team. A key principle of co-production is prioritising relationships, so developing the team and designing foundational values has been important.
In co-production, the people or communities who are likely to benefit from the project need to be involved all the way through, and their lived experiences valued. To keep the MasterChef kitchen image going, before we could start making any afternoon tea items, we need to know who’s going to be eating the afternoon tea. Then we can make sure we meet any requirements they have, and use what’s important to them to help make the decisions we make.
So far in The Co-production Project, we’ve spent quite a lot of time talking to women about what’s important to them when it comes to women’s health. We connected with these women through the charity Good Bitches Baking, who I already had a relationship with. These women are volunteers for the charity, whose aim is to bake the world a better place.
Through conversations with this community we have been able to start considering areas for women’s health that research questions can be developed about with their input. Fittingly, we’ve mostly talked over a cuppa and cake. This more informal style helps us to take the time to build connections and listen to their experiences.
I wouldn’t say we’ve got a test kitchen kitted out yet, nor have we figured out an ingredients list. But we are getting more comfortable at sitting with the discomfort of having no recipe to follow.
Anjuli Muller is a PhD student and TPM Whānau member exploring co-production and participatory public engagement through the subject of women’s health in Aotearoa New Zealand.
Jean Donaldson is a designer and native bird fanatic based in Te Whanganui-a-Tara. You can see more of her work at https://jeanmanudesign.com/.